MRI Machine – The Marvel of Modern Physics and Engineering
Just recently, researchers have discovered cancer was probably around in ancient Egypt, and people were also looking for treatment options, that’s much longer than previously thought. Scientists found two human skulls from ancient Egypt with cut marks around lesions that are believed to have come from attempts to treat cancer. One skull dates back more than 4,300 years, and the other is more than 2,300 years old.
Thinking about treating cancer today, chemotherapy, radiation, and even transplant surgery probably come to mind. However, these treatments are for very serious cases, and they are unbearable for some people. We can avoid these torturing methods by detecting the illness before it gets to damaging stages.
In today’s world, people of all ages have the ability to identify the causes of aches and pains in their bodies. Detecting muscle tears, cancerous tumors, traumatic brain injuries, or even pinpointing infections before they develop has become commonplace in the 21st century. This ability is thanks to a relatively recent discovery: the MRI (Magnetic Resonance Imaging) machine.
A Brief History – How Did MRIs Come to Be?
Before the advent of the MRI, Professor Wilhelm Röntgen discovered what we now know as the X-ray. An X-ray, simply put, is an image of the inside of an object, typically the human body, created using high-energy electromagnetic radiation with short wavelengths that can penetrate various materials.
If you’ve ever broken a bone, you’ve likely had an X-ray taken. Röntgen, a professor in Germany, observed a fluorescent glow of crystals on a table near his cathode ray tube. This discovery captured the attention of scientists worldwide and was eventually used on battlefields by physicians to locate bullets and treat wounded soldiers.
After X-ray, ultrasound emerged as the next significant advancement in the medical field. Ultrasounds were found to be invaluable for imaging blood flow in the heart. While both X-ray and ultrasound machines were critical medical tools at the time, neither could look inside the body without causing harm.
Then came the birth of MRI (Magnetic Resonance Imaging). This innovation is thanks to Raymond Damadian, a medical doctor and research scientist, who developed the use of magnetic resonance imaging as a tool for medical diagnosis.
Dr. Raymond Damadian, born on March 16, 1936, in New York City, earned his bachelor’s degree in mathematics from the University of Wisconsin-Madison in 1956, followed by a medical degree from Albert Einstein College of Medicine in 1960. After meeting his wife Donna Terry and marrying her upon completing medical school, they had three children. Damadian became interested in detecting cancer, motivated by the memory of his grandmother’s painful death from breast cancer in 1969.
In 1970, Damadian proposed the idea for the first whole-body MRI scanner. He was able to differentiate between cancerous and non-cancerous cells, leading to a significant breakthrough in medical imaging. In 1974, he received a patent for his MRI technique.
After years of extensive trial and error, Damadian performed the first full-body scan of a human being in 1977 to diagnose cancer. This year marked the fruition of the full-body MRI as we know it, with Damadian naming his invention the “Indomitable.”
Unfortunately, Damadian’s design was deemed ineffective for clinical purposes due to being extremely slow and leaking excessive amounts of expensive chemicals. British scientist Peter Mansfield refined these earlier findings, developing them into the rapid imaging technology that is now a cornerstone of modern medicine.
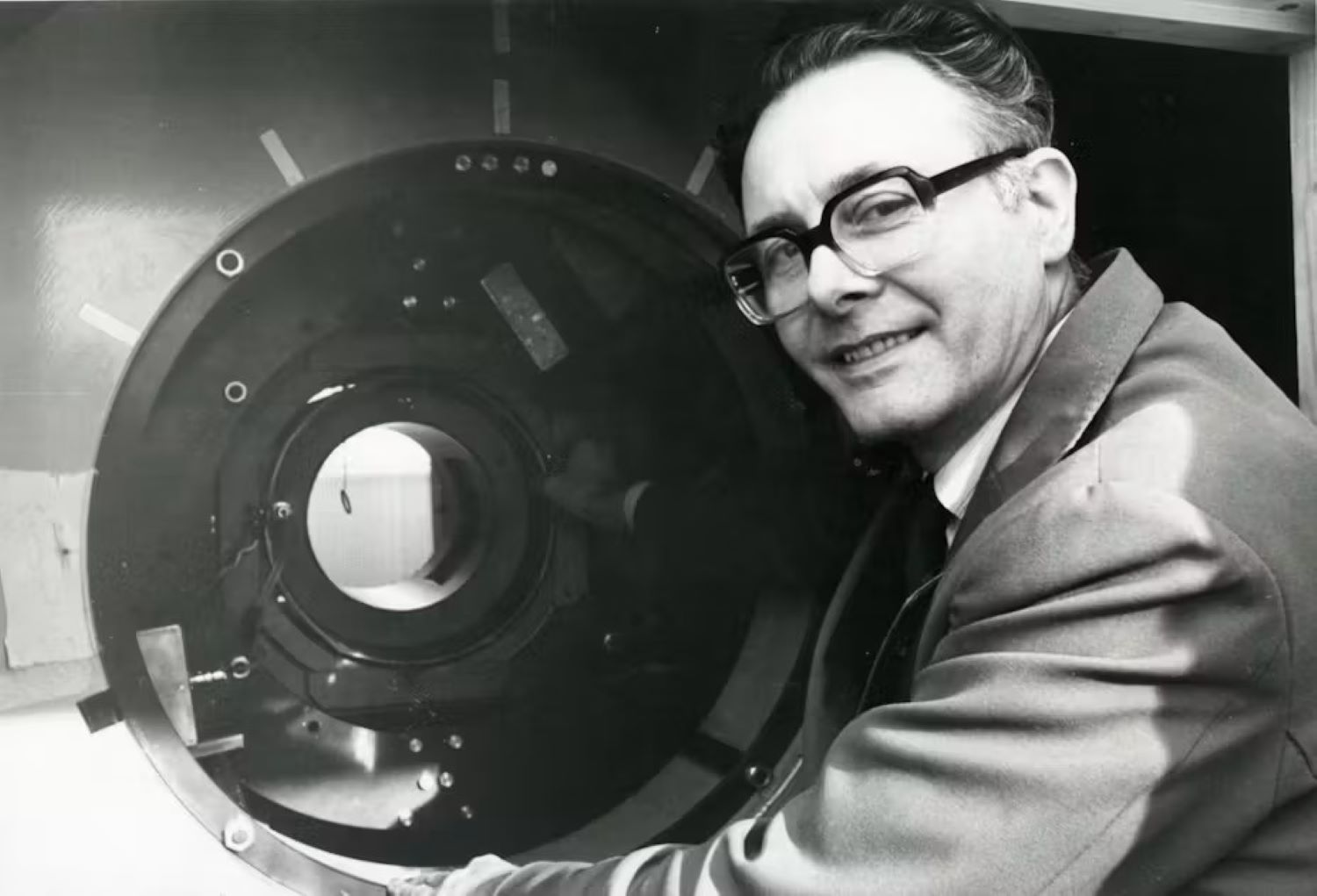
MRIs today are adept at detecting conditions like osteoporosis in bones and pelvic joint issues that X-rays cannot detect. They assist in diagnosing various potentially serious conditions, including disorders of the brain, spinal cord, and liver.
MRIs also help identify issues related to female reproductive organs and can pinpoint areas with bleeding or infections with their ability to visualize deep inside the body. They are commonly used to identify tumors or small fractures that X-rays may miss.
The Fundamental – How Does MRI Work?
Our bodies are made up of 60% water, and each of the billions of water molecules inside us consists of an oxygen atom bonded to two hydrogen atoms, known as H₂O. Small parts of the hydrogen atoms act as tiny magnets and are highly sensitive to magnetic fields. Water itself is magnetic.
An MRI scanner is one of the primary diagnostic tools that doctors use to examine the inside of our bodies. The first step in taking an MRI scan involves using a large magnet to create a uniform magnetic field around the patient. This magnetic field is then adjusted with gradients into smaller sections of varying magnetic strengths to isolate specific body parts, such as the brain.
Normally, the water molecules inside us are arranged randomly. However, when we lie inside a magnetic field, most of our water molecules align their movements to synchronize with the field’s rhythm or frequency. The water molecules that do not synchronize with the magnetic field are called low-energy water molecules.
In an MRI machine, radio waves are used to interact with the low-energy water molecules that are not aligned with the magnetic field. This interaction allows the machine to create an image of specific body parts, such as the brain, by detecting the signals emitted from these molecules. The radio waves move at the same rhythm or frequency as the magnetic fields generated by the MRI machine.
When radio waves that match the magnetic field are sent out, the low-energy water molecules absorb the energy needed to align with the magnetic field. Once the machine stops emitting radio waves, these molecules release the absorbed energy and return to their original positions.
The MRI machine detects this movement and sends the signal to a powerful computer. Using specialized imaging software, the computer translates this data into a detailed image of the scanned body part, such as the brain.
By capturing images of the body in each section of the magnetic field, the MRI machine constructs a final three-dimensional image of the organ. Doctors can then analyze this detailed image to make precise diagnoses and develop effective treatment plans.
The Marvel Engineering of MRI – How Has Modern MRI Evolved?
Despite their futuristic technology, early MRI machines couldn’t achieve the results that modern ones can. To harness the full potential of these machines, physicists and engineers had to first delve into and master the principles of quantum mechanics, superconducting magnets, computer science, and mathematics.
Achieving Intense Magnetic Field with Superconductors
MRI machines use coils to manipulate and detect signals from hydrogen atoms in the body. These coils are placed inside the machine’s strong magnetic field. They send out precise radiofrequency pulses that disrupt the alignment of hydrogen atoms, causing them to emit signals as they realign.
At the same time, these coils also act as receivers, picking up these emitted signals. Advanced MRI machines often use separate coils for transmitting and receiving signals. These coils are positioned closer to the body to improve the strength of the signals and the quality of the images produced.
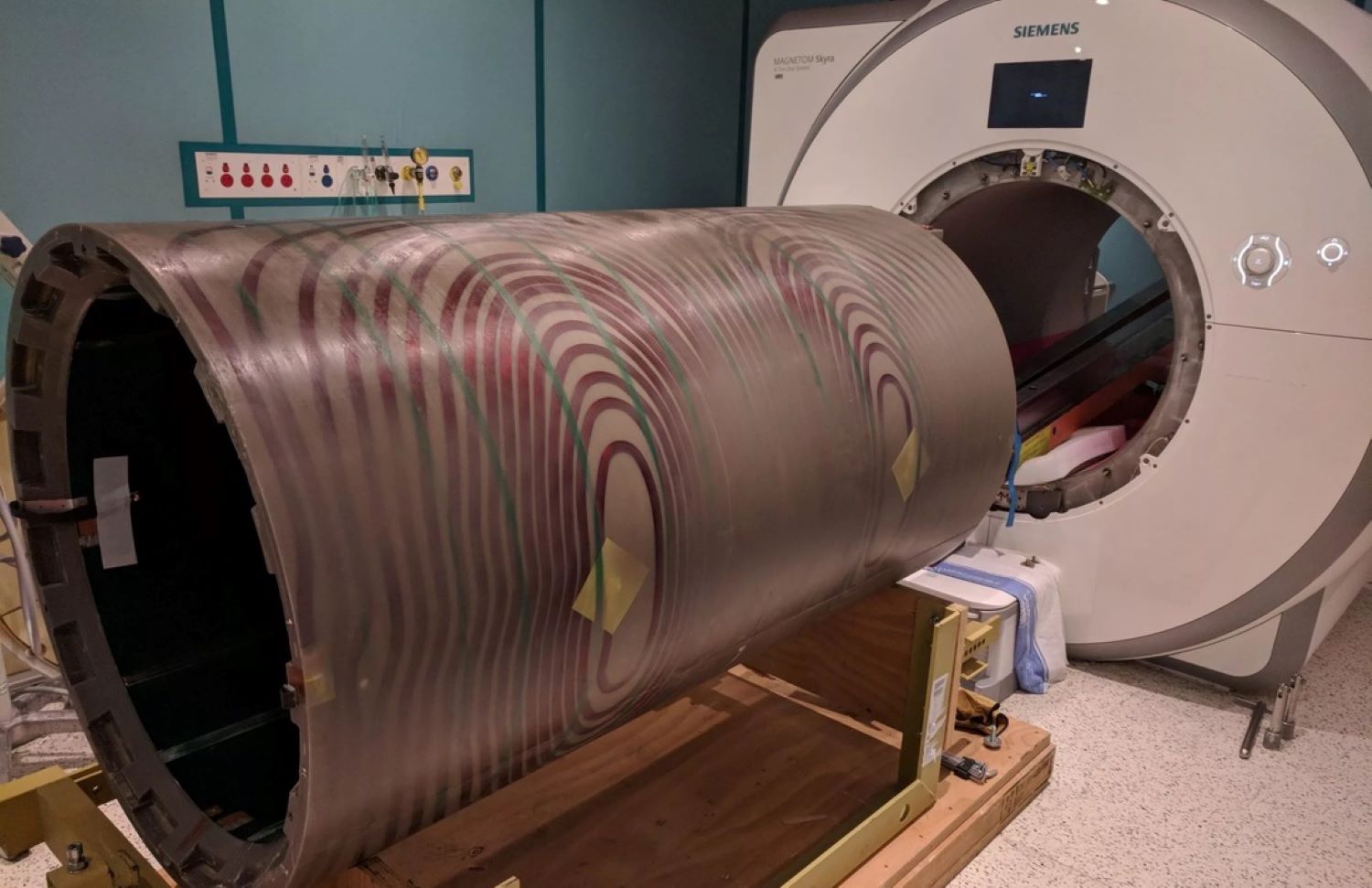
We can increase the number of hydrogen atoms aligning with the MRI’s magnetic field by making the field stronger. This alignment allows the hydrogen atoms to produce a stronger signal as they realign.
Simply boosting the MRI’s magnetic field strength enhances the signals collected, improving image quality. MRI machines typically operate at field strengths of 1.5 to 3 tesla, much stronger than the Earth’s magnetic field or a fridge magnet. These fields are powerful enough to lift nearby wheelchairs off the ground. For advanced research, MRI machines can generate even stronger magnetic fields, up to 20 teslas, which is incredibly difficult to achieve.
Early MRI machines used permanent magnets but were limited to weaker fields, around 0.5 tesla, which affected image resolution. Electromagnets were later used to achieve stronger fields, but standard ones couldn’t reach the necessary strength of 1.5 tesla without overheating ordinary wires. Engineers then developed superconducting coils, which can carry high currents needed for strong magnetic fields without heating up.
Superconductors are a remarkable technology where temperature plays a crucial role. Unlike regular metals whose resistance decreases as they get colder, superconducting materials reach zero resistance at temperatures close to minus 273 degrees Celsius, or absolute zero. This unique property allows electric currents to flow indefinitely in a loop of superconducting material without any need for a power source.
In practical terms, the main superconducting coil in MRI machines operates without consuming direct electrical power. Instead, energy is primarily used to cool the coil to maintain its superconducting state, effectively keeping the MRI magnet running continuously. The energy required to operate an MRI for a year is equivalent to that used by 25 four-person households, totaling around 130,000 to 140,000 kWh annually.
The most commonly used superconducting material in MRIs is niobium-titanium (Nb-Ti). Due to the high demand for high-resolution medical imaging, approximately 80% of all extracted Nb-Ti is used in MRI machines. Achieving the extremely low temperatures required for superconductivity involves using a highly efficient refrigerant.
Cooling System – Keeps the Helium in its Liquid Phase
In the early days, MRI machines cooled their superconducting wires by submerging them in a bath of liquid helium. About one thousand liters of liquid helium at minus 269 degrees Celsius were poured into the machine to bring the superconducting coil as close to absolute zero as possible.
As the helium evaporated, it escaped the machine as a gas, necessitating regular refilling of liquid helium. Despite helium being abundant in the universe, its lightness allows it to escape Earth’s atmosphere and dissipate into space. To obtain helium, we extract it from underground gas caverns where it accumulates as a byproduct of uranium and thorium radioactive decay.
However, once helium escapes into the atmosphere, it is lost forever, rising to the upper atmosphere and being gradually swept into space by solar winds. This depletion of natural helium resources poses a significant concern for future availability. The traditional method of cooling MRI machines using liquid helium was costly and unsustainable, requiring up to $26,000 annually for helium refills.
To address this issue, modern MRI machines utilize vacuum-sealed chambers to contain the liquid helium without allowing it to evaporate. This innovative design eliminates the need for refills and significantly reduces operational costs. These advanced “zero boil-off” machines have become standard in MRI technology, ensuring efficient and environmentally sustainable operation.
Modern MRI machines employ an electric refrigerant cycle to maintain the helium in its liquid phase and keep the magnets sufficiently cool to sustain their superconducting state. This cycle efficiently regulates temperature within the MRI system, ensuring optimal performance and longevity of the equipment.
Real-time Magnetic Resonance Imaging (RT-MRI)
Researchers have unlocked the ability to conduct real-time scans and observe activities like speaking, singing, and swallowing in action. They have also developed techniques to use MRI for visualizing genetic expression in the brain during learning.
Real-time magnetic resonance imaging (RT-MRI) allows for imaging dynamic processes as they occur, without the need for repetition or synchronization. This capability is facilitated by advancements in modern MRI technology, such as fast-switching gradients and parallel imaging techniques.
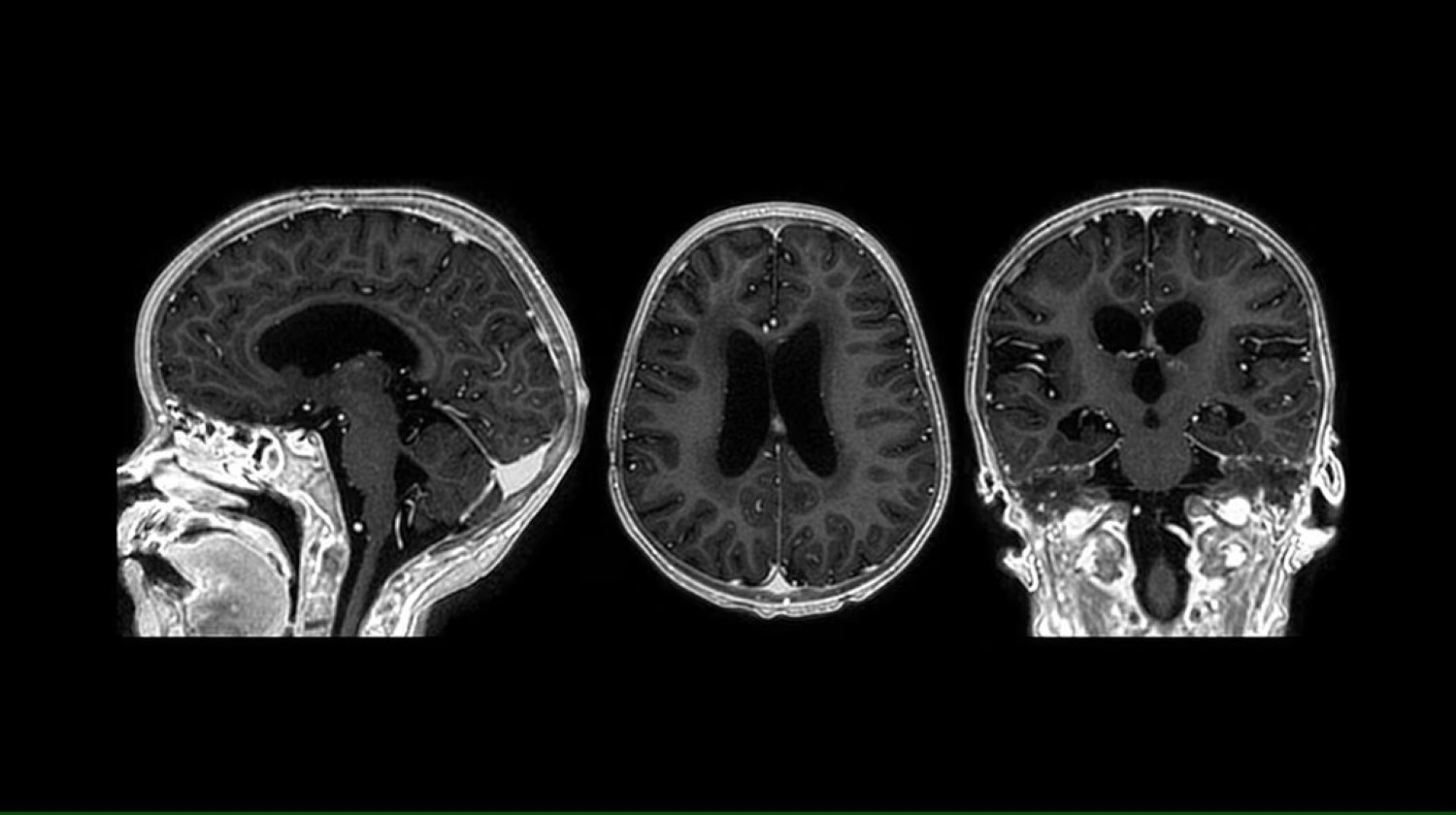
Real-time MRI (RT-MRI) is compatible with many, though not all, MRI sequences, including spoiled gradient echo, balanced steady-state free precession, and single-shot rapid acquisition with relaxation enhancement.
RT-MRI has become essential in both diagnostic imaging and guiding invasive procedures. Its unique diagnostic value shines in areas of the body that experience significant and often irregular motion, such as the heart, gastrointestinal system, upper airway vocal tract, and joints. It is also highly valuable in interventional procedure guidance, especially for procedures that need multiple types of soft-tissue contrast and flow information.
The Quest to Make MRIs More Accessible
If your doctor orders an MRI, it can seem intimidating, even before you think about the cost. MRIs can range from free, with the right insurance, to over $8,000 without insurance. The main factor affecting the cost is whether you have insurance, are on Medicare, or are uninsured.
A standard MRI scanner uses a large, powerful superconducting electromagnet, which drives the machine’s cost to $1.5 million or more, making MRI scans inaccessible to 70% of the world’s population. Even in the United States, getting an MRI might involve days of waiting and a long drive to a distant hospital at an odd hour.
MRIs are constantly evolving, and the core technology is starting to branch off. Researchers want the highest resolution and strive to increase the strength of the magnet, while some hospitals and companies are making them smaller and cheaper, as more practical and inexpensive MRIs are incredibly useful in the field. Talking about making MRIs more accessible, we need to talk about Hyperfine.
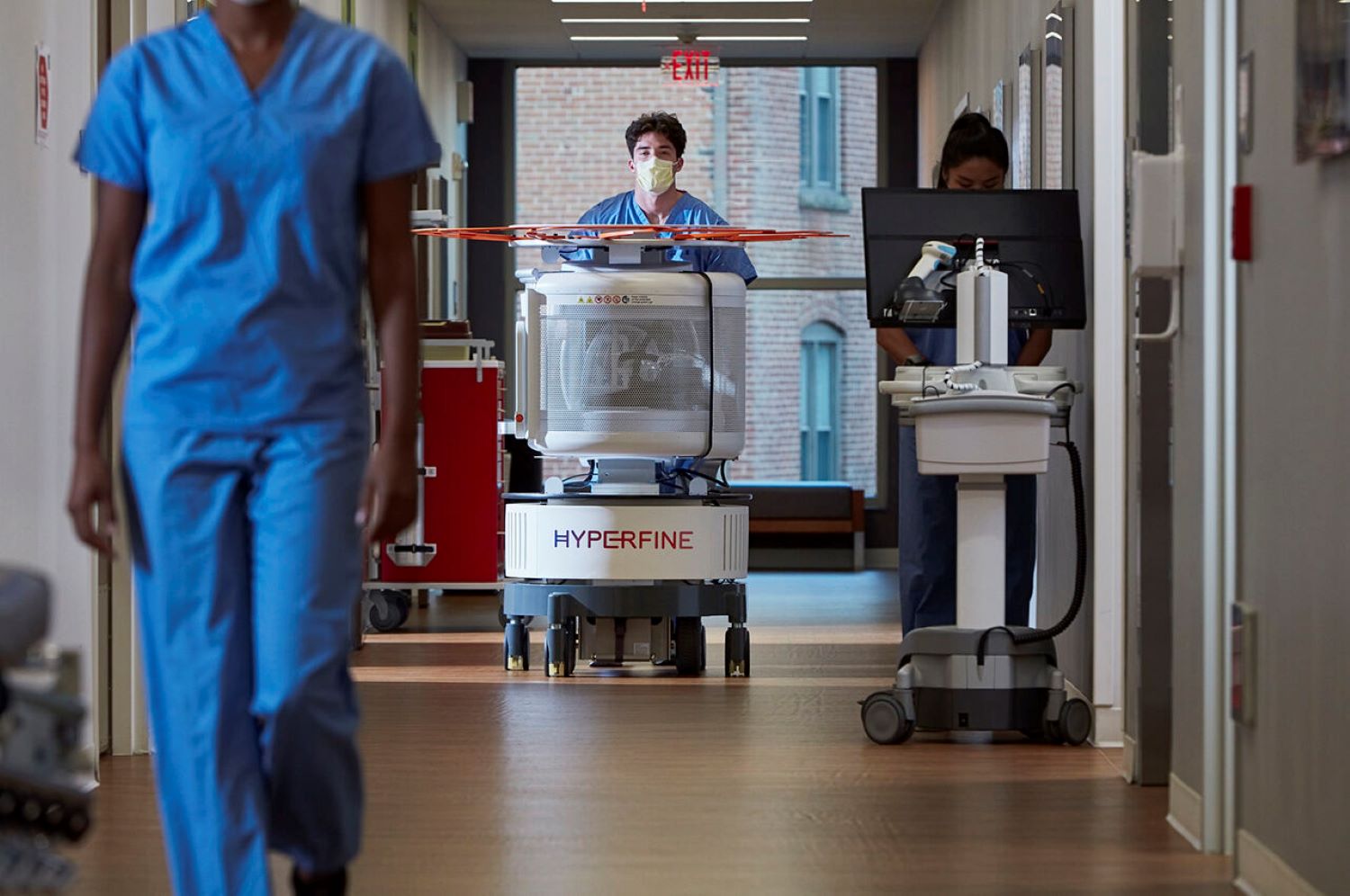
Hyperfine has revolutionized medical imaging with its innovative Swoop, a portable MRI scanner that marks a significant departure from traditional MRI technology. The Swoop’s groundbreaking design features a small permanent magnet, a stark contrast to the large superconducting magnets found in conventional MRI machines.
This technological leap allows the scanner to be wheeled directly to a patient’s bedside, providing critical imaging without the need for patients to be moved to dedicated imaging suites.
In August 2020, Hyperfine achieved a major milestone when the Swoop became the first low-field MRI scanner to receive FDA approval for brain imaging. This regulatory clearance underscored the scanner’s reliability and safety for clinical use, particularly in environments where mobility and rapid diagnostic capabilities are paramount.
Institutions like Yale New Haven Hospital have integrated the Swoop into clinical studies, demonstrating its utility in scenarios where patients are too critically ill or unstable to undergo imaging in traditional MRI or CT machines.
“We’re not trying to replace high-field scanners,” explains Jonathan Rothberg, founder of Hyperfine. “Instead, we’re expanding the accessibility of MRI technology by making it more affordable and convenient.” The Swoop operates at a field strength of 64 millitesla, significantly lower than traditional MRI machines which can reach several tesla.
This lower field strength impacts image resolution but allows for portability and cost-effectiveness, opening up new possibilities in emergency medicine and intensive care units.
Looking ahead, Hyperfine is committed to advancing the Swoop’s capabilities further. They are exploring enhancements in image quality through advanced signal processing techniques, including artificial intelligence.
These innovations aim to overcome the resolution limitations associated with low-field MRI, ensuring that the Swoop remains at the forefront of accessible and effective medical imaging solutions globally.
The Promising Future of MRIs
According to Brad Sutton, a professor of bioengineering at the University of Illinois Urbana-Champaign and the technical director of the Biomedical Imaging Center at the Beckman Institute, in the next few years, we will see new MRI systems with even higher magnetic fields, providing even higher spatial resolution images of the body and brain.
He said, “At the same time, we are also seeing new MRI systems that are small and portable, which can be placed in the doctor’s office for easy access. We will see systems that integrate information across all patients to better understand what we are seeing in the image and what it means for the health of the patient.”
He added, “We will also see new information when looking at the images, with new techniques leading to images where the signal intensity in the image represents quantitative information about the status of the tissue, including concentrations of key molecules in each pixel of the image, mechanical and electrical properties of the tissues, information about how the brain is performing its activities including changes to the tissue structure and genetic expression, and the systems will produce actionable 3D visualizations of the person in the scanner so that a doctor can perform virtual interventions and virtual surgeries to see the best way to treat the patient.”
“Given the pace of development from when Paul Lauterbur imaged his first living sample (a clam) until now, I am certain that we will see these developments before another 50 years,” stated Brad Sutton.